As humans transitioned from nomadic wanderers to cultivating the land and tending herds, they engaged with unintentional experiments. You might envision fields of early grains swaying in the breeze or small flocks of the first domesticated animals, and you’d be right. But within these seemingly simple acts of survival lay the seeds of a profound scientific understanding: the passing of traits from one generation to the next – what we now call inheritance.
In biology, inheritance refers to the passing of genetic traits from parents to offspring. This process, also called heredity, explains why offspring resemble their parents and why there's variation within a species. Inheritance is driven by the transmission of genetic information, primarily in the form of DNA, from one generation to the next
Consider the patient work of early farmers selecting the best seeds for the next planting. They noticed that certain characteristics – the size of the grain, the sweetness of the fruit – tended to reappear in the offspring. This wasn’t a conscious application of genetic laws, yet it was a practical recognition that “like begets like,” albeit with variations that could be influenced by their choices. You might have experienced this yourself, noticing how seeds saved from a particularly productive tomato plant often yield similar results.
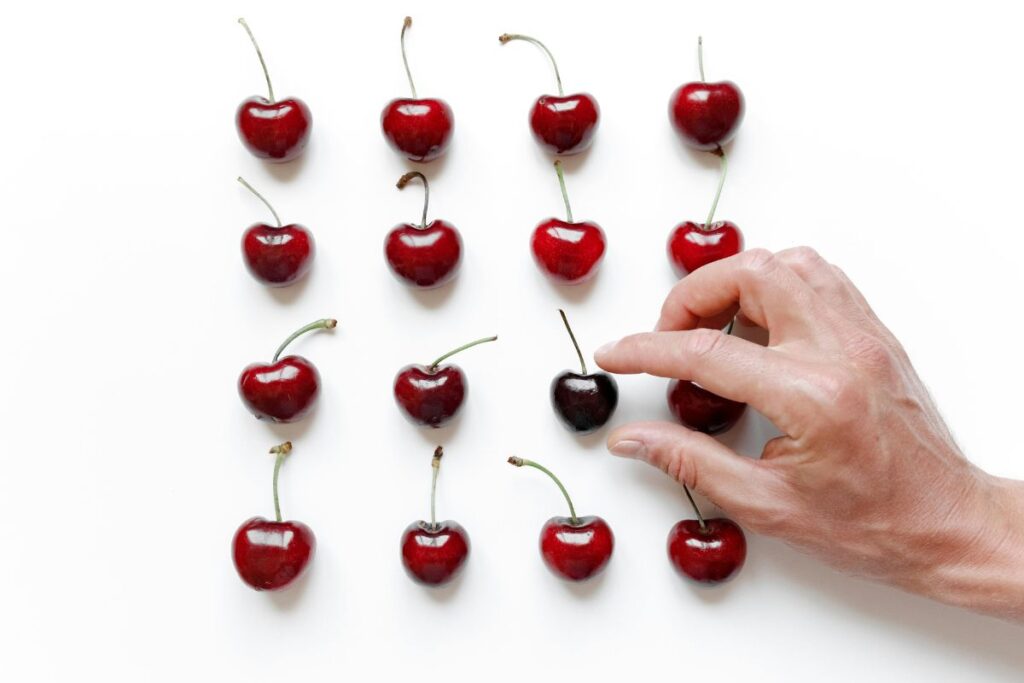
Similarly, the domestication of animals involved a keen eye for heritable traits. Early herders would select animals with desirable qualities – a calmer temperament in goats, a thicker fleece in sheep – for breeding. This wasn’t driven by knowledge of chromosomes or genes, however, it demonstrated an intuitive understanding that these characteristics could be passed down, shaping the traits of future generations. Think of the distinct breeds of dogs, each developed over centuries through the selective breeding of animals with specific desired traits.
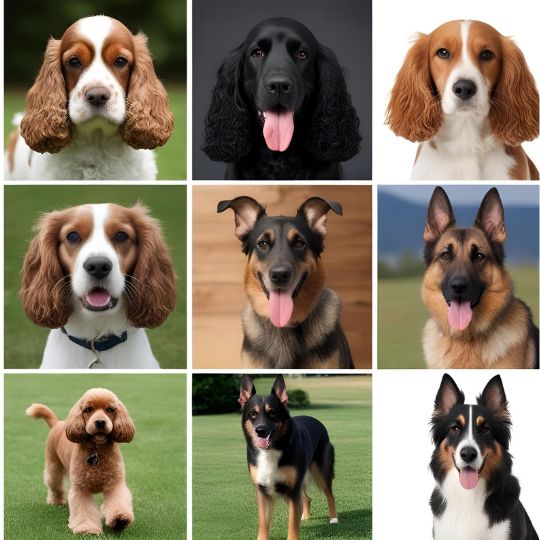
You might be thinking that this early understanding was purely observational, lacking any real explanation for how these traits were inherited. And you’re correct. For millennia, humans manipulated inheritance without knowing the underlying mechanisms. But these consistent observations and the ability to predictably alter the characteristics of plants and animals through selective breeding laid the crucial groundwork for later scientific inquiry. This practical knowledge, accumulated over generations, posed a fundamental question that eventually scientists like Gregor Mendel would attempt to answer.
The Principles of Inheritance (Mendelian’s Genetics)
Okay, let’s delve deeper into Gregor Mendel’s pivotal contributions, drawing from what we know about Mendel’s persistent experiments over 7 years (1856 to 1863) and the significance of his laws within the broader context of inheritance, including the insights gleaned from early agriculture.
Mendel’s meticulous work with pea plants (Pisum sativum) provided the foundational principles for our modern understanding of inheritance. Mendel conducted these experiments with a curiosity to unravel how traits were passed from one generation to the next. His choice of the common pea plant was strategic. Mendel could easily control fertilization through manual pollination, allowing for both self-fertilization and cross-fertilization between carefully selected plants. This level of control was crucial for establishing clear parental lines and tracking the inheritance of specific traits.
Before initiating his controlled crosses, Mendel dedicated two years to observing pea plant forms and their offspring through self-fertilization. This crucial preliminary step ensured the “purity” of his parental lines. By repeatedly selfing plants (when pollen was taken from the same flower, hence called self-fertilization)and confirming that their outward, measurable characteristics remained constant across generations (e.g., tall plants consistently producing tall offspring), Mendel established what we now term homozygous lines for the traits he was interested in. Then, Mendel tracked seven distinct characteristics, each with two clear and contrasting forms: height (tall/short), pod shape (inflated/constricted), seed shape (smooth/wrinkled), pea color (green/yellow), and others. Through this rigorous preparation, Mendel ensured he was starting with genetically consistent parental stock, a critical element for discerning clear patterns of inheritance.
This was, undoubtedly, a prolonged and systematic approach conducted by Mendel that marked a departure from the more observational studies of inheritance prevalent at the time. Mendel’s methodology involved not only crossing pure-breeding parents but also meticulously tracking the inheritance of traits through subsequent hybrid generations (F1, F2) and backcrosses to the parental lines (B1, B2). These controlled crosses allowed him to generate genetically hybrid populations and observe the patterns of trait expression across multiple generations.
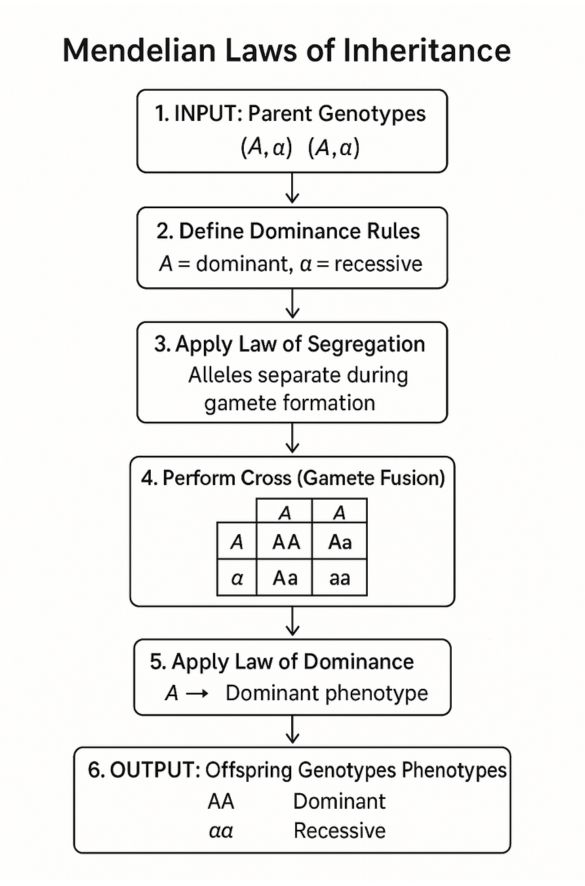
A key insight from Mendel’s early crosses challenged the prevailing “blending” theory of inheritance. When Mendel cross-pollinated purebred plants with contrasting traits (e.g., smooth seeds x wrinkled seeds), the F1 generation did not exhibit an intermediate blend of the traits. Instead, all the offspring displayed the phenotype of only one of the parents. Mendel termed the expressed parental trait the dominant trait, and the trait that seemed to disappear in the F1 generation he called the recessive trait. From this observation, Mendel proposed his principle of uniformity, stating that the progeny of a cross between purebred parents differing in a single trait would be uniform and resemble only one parent. There are some exceptions to this principle, such as incomplete dominance and codominance, which were discovered after Mendel’s time.
To investigate whether the recessive trait was truly lost in the F1 generation or merely hidden, Mendel performed a crucial second step: he allowed the F1 hybrid plants to self-fertilize, producing the F2 generation. This monohybrid cross, focusing on a single trait, yielded a remarkable result. The recessive trait reappeared in the F2 generation. Moreover, Mendel observed a consistent 3:1 ratio of the dominant to the recessive phenotype in the F2 offspring. The importance of multiple trials and large sample sizes showing a quantitative pattern is a hallmark of Mendel’s scientific rigor.
While rejecting the blending theory, Mendel hypothesized that each parent contributed “particulate matter” (which he called “elementen,” and we now know as alleles) to their offspring. For each trait, an individual carried two of these elementen. Mendel’s brilliance is evident in his notation, using capital and lowercase letters (e.g., Aa) to represent the hybrid genotype, reflecting the two different alleles of a gene.
Did you know? Gregor Mendel was not only the father of genetics, but he was also a passionate beekeeper and made significant contributions to meteorology. He was the first to scientifically describe a tornado in Brno in 1870.
The reappearance of the recessive trait in the F2 generation and the consistent 3:1 ratio led Mendel to his principle of segregation. This principle states that the two alleles for a heritable character separate (segregate) during gamete formation (meiosis), and each gamete carries only one allele for each trait. Thus, meiosis produces equal numbers of gametes carrying each allele. This segregation of alleles during gamete formation explains why the recessive trait, though hidden in the F1 generation (carrying one dominant and one recessive allele), could reappear when two F1 individuals (each carrying one recessive allele) produced offspring.
Beyond single-trait inheritance, Mendel also investigated the inheritance of two traits simultaneously through dihybrid crosses. As he crossed purebred plants differing in two traits (e.g., seed color and seed shape) and then crossed the resulting F1 dihybrids with each other. Based on his principle of segregation, Mendel predicted that the alleles for different traits would sort into gametes independently of one another. His results from the dihybrid cross (315 round yellow, 108 round green, 101 wrinkled yellow, 32 wrinkled green), supported his principle of independent assortment. This principle posits that the inheritance of one trait does not affect the inheritance of another trait. We even know that this holds true for genes located on different chromosomes.
Parent 1 | Parent 2 | B | b |
B | BB | Bb |
b | Bb | bb |
In essence, early agriculture and domestication represent humanity’s first practical engagement with inheritance, demonstrating the “what” of passing traits down. Mendel’s work, centuries later, began to unlock the “how” by establishing the fundamental rules governing this process. His laws provided the intellectual bridge between the ancient observations of farmers and the modern understanding of genetics rooted in DNA.
Therefore, while Mendel’s specific experimental methodology differed greatly from the trial-and-error of early agriculture, the phenomenon he studied was the same, making his work a crucial step in our long journey to understand the blueprint of life.
So, the next time you see a farmer tending their crops or a breeder caring for their animals, remember that they are participating in a tradition that stretches back millennia ─a tradition that not only sustained civilizations but also provided the initial, practical insights into the fundamental principles of inheritance, insights that would eventually blossom into the science of genetics we know today, thanks in no small part to the groundbreaking work of individuals like Gregor Mendel.